Contact Person: S. Evgenidis [sevgenid@chem.auth.gr], R. Oikonomidou [rania.oik@gmail.com].
The Multiscale Boiling Experiment is a fluid science experiment developed and built by Airbus for the European Space Agency (ESA), to address the fundamentals of boiling in microgravity. The supply mission (CRS-18, Figure 1) has been launched twice from Cape Canaveral, Florida on board the International Space Station (ISS), at 2019 and 2020. This experiment is operated and controlled by the Belgian User Support and Operation Centre (B-USOC) in Brussels.
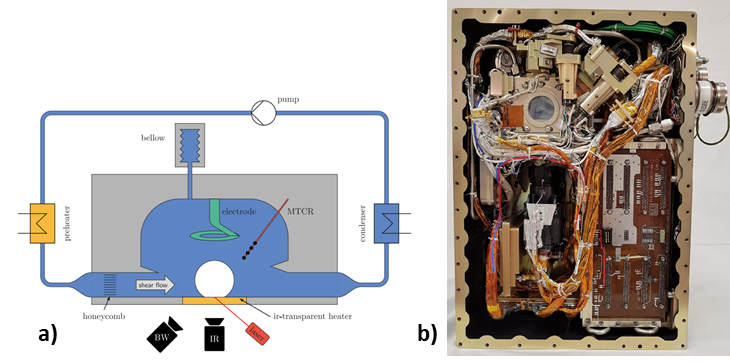
Figure 1. a)Schematic description of the Multiscale Boiling experimental setup (BW: Black and White camera, IR: Infrared camera, MTCR: micro-thermocouple rack), b) Image of the Multiscale Boiling Experimental setup
The Multiscale Boiling Experiment investigates the growth of vapor bubbles during subcooled boiling in microgravity, under or in the absence of shear flow and electric field forces. The absence of gravity allows studying in depth boiling principles as it cancels the transfer of heat through natural convection and it elucidates the impact of surface tension and pressure forces on bubble growth. Moreover, it hinders bubble detachment from the nucleation site and thus allows prolonged examination of bubble growth phenomena. The application of external forces determines the shape of the bubble (Figure 2). In the absence of external forces (pool boiling) bubbles are spherical. Shear flow tilts the bubble towards the flow direction and slightly deforms its spherical shape. Under electric field, bubbles are elongated towards the vertical direction and become ellipsoidal. The growth rate of boiling bubbles depends on the applied experimental parameters such us pressure, initial subcooling liquid temperature, heat flux provided from the heater and waiting time between heater activation and laser pulsation for the nucleation of the first bubble.
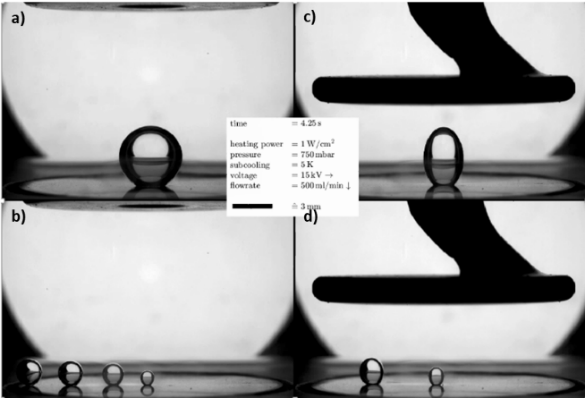
Figure 2. Multiscale Boiling bubbles shape in the case of a) Pool boiling, b) Shear flow, c) Electric field, d) Electric field and shear flow.
Bubble growth is studied by capturing high speed, black and white video images. As it appears, processing these images with conventional image processing algorithms is not feasible. The background lighting of the test cell reflects over the surface of bubbles and makes it difficult to detect the actual bubble boundaries. These light reflections appear at different regions of the bubble contour that vary with bubble size and population. Moreover, the vertical temperature gradient of the liquid close to the heater changes liquid refractive index and distorts the vertical dimension of bubble boundary (refractive image distortion, see Figure 3).
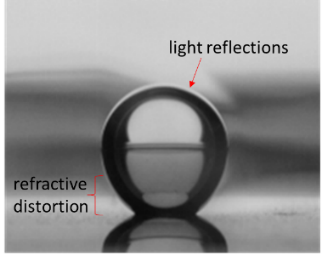
Figure 3. Light reflections around the contour of a pool boiling bubble and refractive optical distortion of the bubble shape close to the heated substrate.
A new specialized image processing algorithm is developed by our research team, in collaboration with the Foundation of Research and Technology in Crete (FORTH) and the Technical University of Darmstadt (TUD), so as to treat both light reflections and refractive optical distortions arising. This algorithm is able to detect the trusted points on the bubble contour with a subpixel accuracy (Figure 4a, 4b). Depending on the type of boiling and the resulting bubble shape, the trusted points are fitted with standard geometrical shapes, i.e. circle, ellipse (Figure 4c). The basic geometrical features of the bubble, i.e. bubble diameter, contact line diameter, contact angles, can be measured by using either the resulting boundary fit or the geometrical fit (Figure 4d).
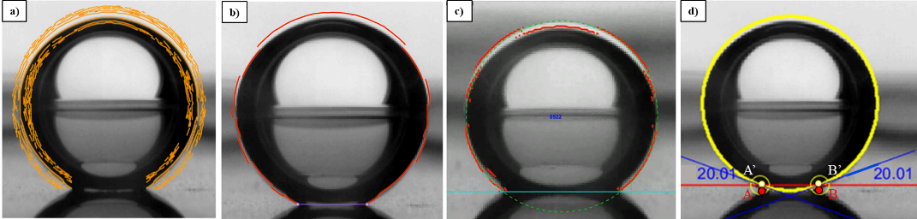
Figure 4. a) Trusted points of bubble contour on the orientation of bubble curvature, b) Distinct segments resulting from the connection of shorter image edges after discarding the overlapped parts of continuous segments, c) Circle fit applied on the trusted points of a pool boiling bubble, d) Contact angles measurement using the circle fit.
Using this algorithm, our research team analyzed all pool boiling experimental runs (160 experimental cases* 3 repetitions) in order to study the effect of each experimental parameter (pressure, heat flux, subcooling temperature, waiting time) on bubble growth rate. Figure 5 shows the effect of boiling conditions on bubble growth.
As shown in Figure 6a, all bubble growth curves (black lines) are accurately fitted with power law equations (yellow dashed lines), corrected by a time shift factor (b) to predict successfully positive bubble sizes at zero time scales. The variation of exponent ‘n’ and coefficient ‘a’ with twait parameter is also described by power law equations, the exponents ‘n1’, ‘a1’ and coefficients ‘n2’, ‘a2’ of which, depend on heat flux, subcooling temperature and pressure and is given in tables (Figure 6 b, c). As an overall, the resulting fitting equation is .
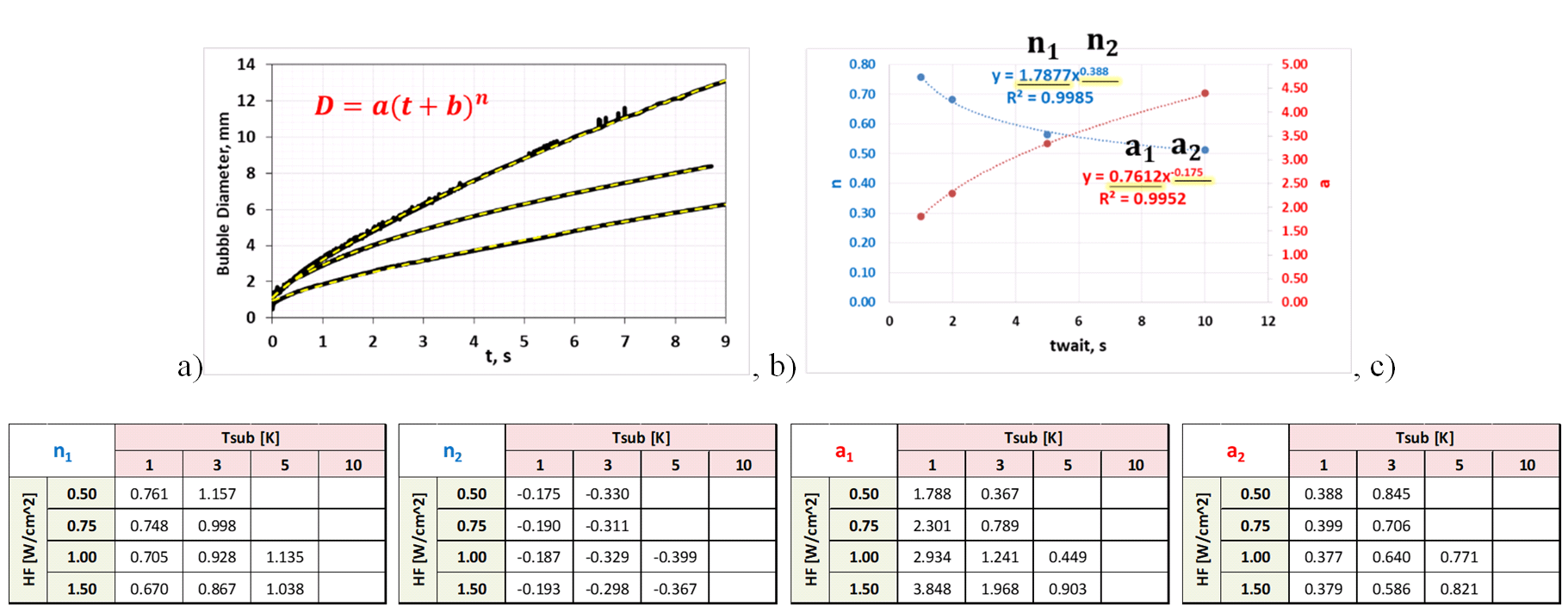
Figure 6. a) Fitting bubble growth curves with power law equatios, b) variation of power law exponent and coefficient with twait parameter discribed by new power law equations, c) dependance of secondary power law exponents and coefficients on the heat flux and subcooling temperature for the case of 500mbar pressure.